The innermost planet, Mercury, may be the smallest in the Solar System, but it’s also home to some big surprises.
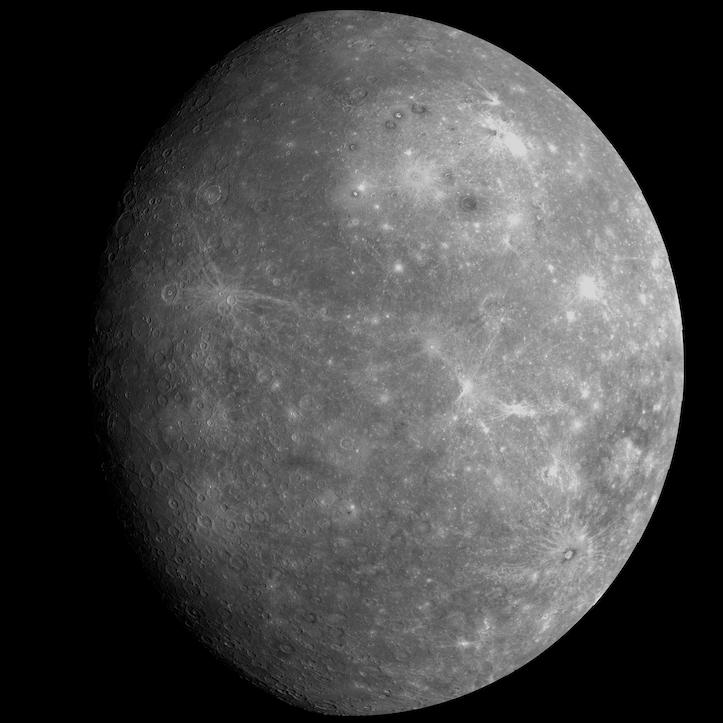
At 4,879 kilometres in diameter, Mercury is smaller than even Jupiter’s moon Ganymede (5,268 kilometres) and its orbit around the Sun is highly elliptical, getting as close as 46 million kilometres, and as far as 69.8 million kilometres. Indeed, it is the most elliptical orbit of all the planets in the Solar System (an eccentricity of 0.21, which renders its orbit more egg-shaped than circular), and it is an orbit that slowly precesses. This means that its closest point to the Sun in its orbit, called perihelion, moves, or precesses, around the Sun with each orbit – think of how a spinning top appears to precess in a circle while it is spinning. In the nineteenth century, classical Newtonian gravity could not explain this precession. It was only with the advent of Albert Einstein’s General Theory of Relativity that it could be explained in the context of the strong gravitational field that close to the Sun.
Mercury takes 88 Earth days to complete one orbit of the Sun, but it spins very slowly about its axis, so that one sidereal day – that it, the time it takes Mercury to complete one revolution of its axis with respect to the fixed stars in the background – is 58.6 Earth days. However, because it spins so slowly, Mercury has gone two-thirds of the way around its orbit by the end of one sidereal day, meaning that the Sun is not in the same position in Mercury’s sky at the beginning of one sidereal day to the next. Instead, the solar day – that is, the time between the Sun being in the same position at the same time – is 176 Earth days. In comparison, Earth’s relatively fast spin means that our sidereal day and solar day are pretty much the same length – sidereal day is 23 hours, 56 minutes and four seconds, and solar day is 24 hours give or take a few seconds at different times of the year because of Earth’s tilt of 23.5 degrees, whereas Mercury has barely any tilt at all.
When we study exoplanets that orbit close to their stars, we find many that are tidally locked. This means that their axial rotation and their orbital period have fallen into sync – a day lasts the same amount of time as a year, which in practice means that as the planet gently rotates it is always showing the same face to its star. This is exactly the reason we always see the nearside of the Moon in the sky.
Mercury is a little different. It’s fallen into sync, but not in a 1:1 resonance. Instead, for every three full rotations on its axis (sidereal days), Mercury completes two orbits of the Sun – a 3:2 resonance. Therefore the Sun does rise and set on Mercury, but very slowly.
During Mercury’s long rotation, temperatures range from a frigid –183 degrees Celsius during the night to a scolding 427 degrees Celsius in the daytime. Yet because Mercury is barely tilted (it leans over by just two degrees relative to the ecliptic plane), the floors of numerous deep craters at the poles are never exposed to sunlight, meaning that ice there remains in a permanent deep freeze, a finding confirmed by NASA’s MESSENGER (MErcury Surface, Space ENvironment, GEochemistry and Ranging) spacecraft that orbited the Mercury between 2011 and 2015.
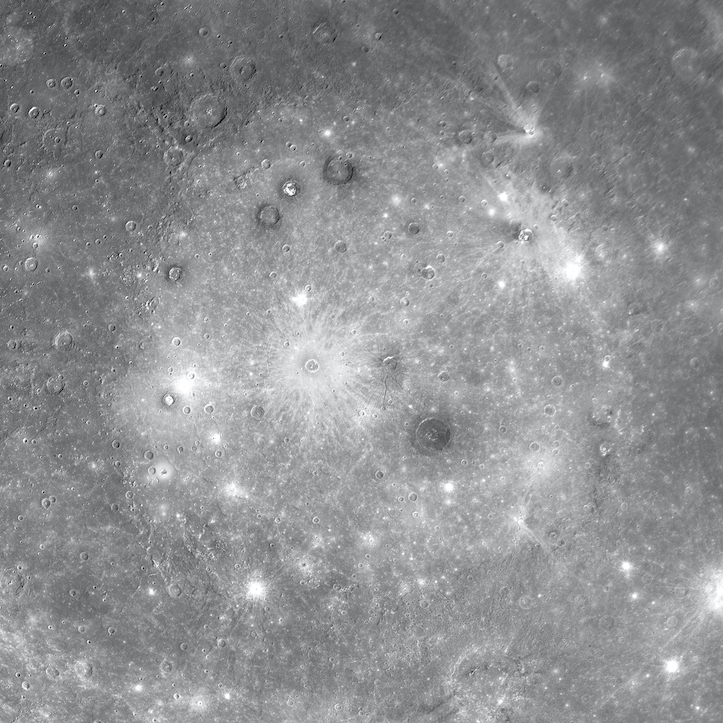
On the surface
It took a long time to get to know Mercury. MESSENGER’s first reconnaissance fly-by of the planet in 2008 (with another fly-by in 2009 before entering orbit) came 33 years after the last spacecraft mission to Mercury, NASA’s Mariner 10, which made three fly-bys of Mercury in 1974 and 1975 and succeeded in imaging just 45 per cent of the planet’s surface.
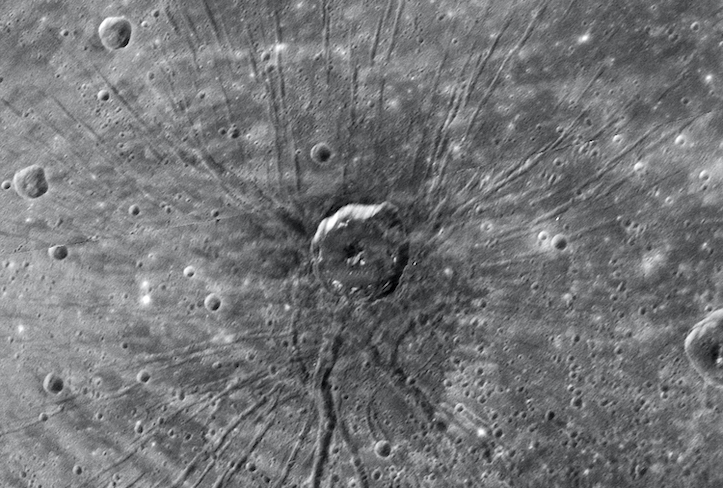
So with over half the planet still to discover, there was much to keep MESSENGER busy. Once in orbit, the spacecraft mapped in high-resolution a surface scarred with impact craters, towering cliffs and sunken trenches, plains of frozen lava and hollow volcanic pits, bright spots, dark rays and downright ‘weird terrain’ (yes, it is called that!), which is a bobbly, disturbed region of the surface that lies on the exact opposite side of the planet to the giant Caloris basin. This is one of the largest impact sites in the Solar System, an enormous crater 1,525 kilometres across, ringed by mountains many kilometres high and dating back to about 3.9 billion years ago, when a 100-kilometre-wide asteroid slammed into Mercury. So violent was the impact that the shockwaves ran around and through the planet, coinciding at the impact’s antipode – directly opposite the impact site on the other hemisphere – where they formed the weird terrain.
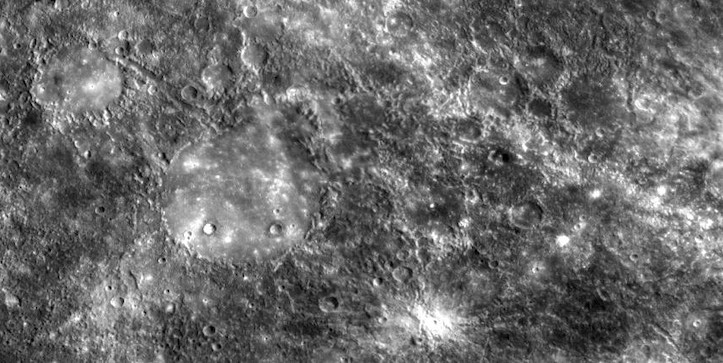
Elsewhere, large expanses of smooth plains dominate the landscape, their very existence a record of periods of intense volcanic activity, with lavas several kilometres thick having poured out from long linear vents to cover the surface and form the plains. Bright markings around craters with steep-walled pits are also interpreted as volcanic deposits from explosive volcanoes, again confirming that volcanism shaped much of Mercury’s crust.
Other irregularly shaped hollows found in craters of all sizes and which appear relatively young may point to Mercury still being alive today. Akin to the ‘Swiss-cheese’ terrain of Mars where carbon-dioxide ice sublimates away causing the surface to collapse into pits, a similar process may be occurring on Mercury. In this case, however, it is volatiles on the planet’s surface such as potassium, rather than ice, which sublimate under the Sun’s intense heat.
Shrinking planet
Mercury has been contracting as its interior has cooled over billions of years. Testament to this global cooling are so-called wrinkle ridges that snake across the planet’s surface, formed as Mercury contracts and the surface buckles up to create these crease-like features, while at the same time already existing valleys begin to sag.
Deep underground, Mercury’s interior contains a great mystery. Its oversized iron core is 3,600 kilometres across, contributing 60 per cent of the planet’s mass and 85 per cent of its volume. The inner part of the core is solid iron, 2,000 kilometres wide, while the outer core remains molten. How did such a small planet come to have such a big heart?
Speculation suggested that perhaps a giant collision in the dim and distant past with a large protoplanet had torn away much of Mercury’s mantle to leave behind a core-dominated planet. However, this theory doesn’t fit the facts. There is no evidence of the whereabouts of the material ripped from Mercury, since Mercury has no moons that could have coalesced from it. Also, MESSENGER has detected relatively large abundances of volatiles on Mercury’s surface. These are materials such as hydrogen that have low boiling points and vaporise easily when subjected to the heat of, say, a giant impact. Such an impact should have removed the majority of volatiles.
Numerous other theories have been put forth, including that the Sun’s magnetic field dictated how much iron and other heavy elements were distributed across the inner Solar System when the planets were forming 4.5–4.6 billion years ago. Grains of iron would have been drawn closer to the Sun by the magnetic field, resulting in them congregating at the heart of the protoplanet that ultimately became Mercury.
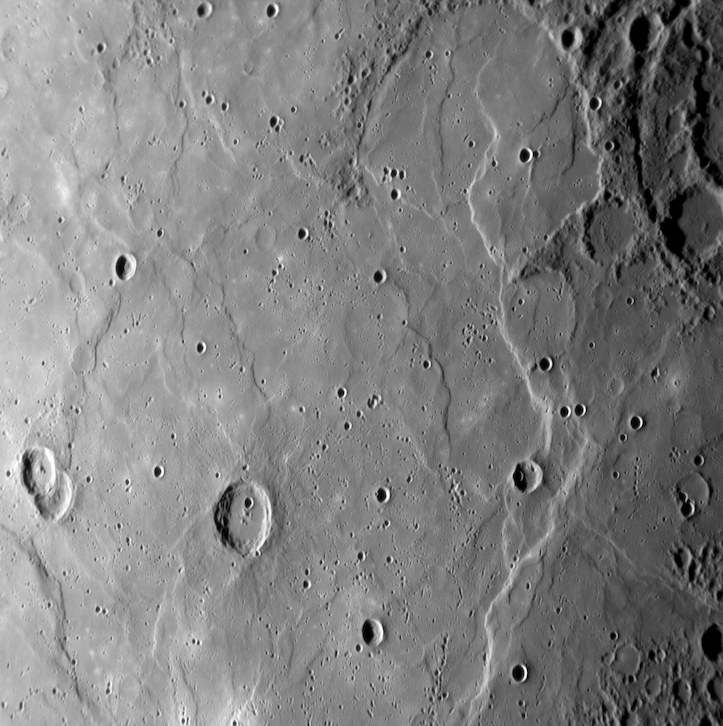
Magnetic personality
Mercury has its own magnetic field. Today, it is just one per cent the strength of Earth’s, but in the past it would have been much stronger. In terms of its structure, Mercury’s magnetic field is similar to that of our planet, with magnetic north and south poles generated by a dynamo produced by a churning molten iron-rich core. However, the magnetic equator on Mercury is offset by about 480 kilometres northward of Mercury’s geographic equator, a geometry that results in a magnetic field about 3.5 times stronger at the north pole than it is at the south geographic pole.
Mercury’s magnetic field is sufficient to deflect the brunt of the solar wind around the planet, creating a magnetospheric ‘bubble’ inside which Mercury is encased. As a result, in the face of the solar wind, which is far more intense at Mercury than farther away at Earth, Mercury is able to cling onto a very tenuous atmosphere – or exosphere – generated and maintained by the interaction of the space environment with the planet’s surface. The solar wind delivers hydrogen and helium, while water vapour is released into the exosphere from comet strikes and sublimation from icy craters. Elements such as sodium, potassium, calcium and magnesium are liberated from the crust during meteoroid strikes, solar wind bombardment or even solar heating; the interactions with the surface ‘sputters’ these species out of the rocks, whereupon they become ionised and swept up in the force lines of the magnetic field. These materials were observed by MESSENGER in a magnetic tail streaming away from the planet, the magnetic field shaped into a teardrop by the force of the gusts of the solar wind blowing past.
MESSENGER was deliberately crashed into the surface of Mercury in 2015, after its fuel was almost depleted. As for future exploration, a European–Japanese mission called BepiColombo launched on 20 October 2018, and is scheduled to arrive into orbit around Mercury in 2025.