
Top Stories
 |
 |
|
|

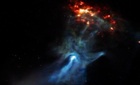

Young pulsar hands
out radiation
...A very young and powerful pulsar, less than 20 kilometres wide, has carved out a beautiful X-ray nebula in the shape of a hand that reaches out across a distance of 150 light years...
read more

Hubble finds hidden exoplanet in archival data
...A powerful image processing technique may allow astronomers to seek out exoplanets that could be lurking in over a decade's worth of Hubble Space Telescope data...
read more

Integral dissects bright gamma-ray burst
...Integral has captured one the brightest gamma-ray bursts ever seen, allowing astronomers to probe the mechanics of the initial stages of such powerful stellar explosions...
read more |
|
|
 |
Spaceflight Now +
 |
 |
|
|

Subscribe to Spaceflight Now Plus for access to our extensive video collections!
How do I sign up?
Video archive

STS-120 day 2 highlights
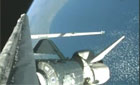 Flight Day 2 of Discovery's mission focused on heat shield inspections. This movie shows the day's highlights.

Play

STS-120 day 1 highlights
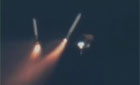 The highlights from shuttle Discovery's launch day are packaged into this movie.

Play

STS-118: Highlights
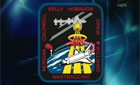 The STS-118 crew, including Barbara Morgan, narrates its mission highlights film and answers questions in this post-flight presentation.

Full presentation
Mission film

STS-120: Rollout to pad
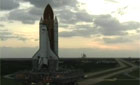 Space shuttle Discovery rolls out of the Vehicle Assembly Building and travels to launch pad 39A for its STS-120 mission.

Play

Dawn leaves Earth
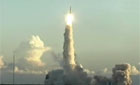 NASA's Dawn space probe launches aboard a Delta 2-Heavy rocket from Cape Canaveral to explore two worlds in the asteroid belt.

Full coverage

Dawn: Launch preview
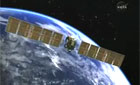
These briefings preview the launch and science objectives of NASA's Dawn asteroid orbiter.

Launch | Science

Become a subscriber
More video
 |
|
|
 |
|
|

What’s the big deal
about dust?
BY KEITH COOPER
ASTRONOMY NOW
Posted: 14 April, 2009
Dust has a habit of getting everywhere, as we all know. It’s even found in space, but interstellar dust is much more important than the fluffy stuff that accumulates on your sideboard at home. Without interstellar dust, you and I and planet Earth wouldn’t even exist.
Star stuff
Stars are alchemists. The temperatures within are high enough to fuse primordial hydrogen into helium, and eventually grow hot enough to fuse helium atoms into a succession of elements including beryllium, carbon, nitrogen, oxygen, and so on. This act of stellar alchemy is known as nucleosynthesis, and when the stars die their embers, in the form of these elements, are spread back out into interstellar space, enriching the chemistry of our Galaxy.
And this is from where we get interstellar dust, but it doesn’t come out of the stars fully formed. Stars have two possible end-points: they swell up into a red giant, before casting off their outer layers, or they explode in a supernova. Both have huge outflows, either from fierce stellar winds of radiation lifting the gaseous envelope off a red giant, or from the energetic blast wave of a supernova ripping the star to shreds. The type of outflow, and the type of star that created it, decides what kind of dust is produced.
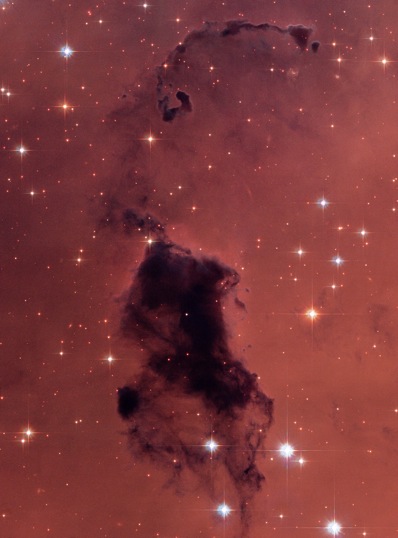
Nearby dust clouds in our Galaxy. Image: NASA/ESA/Hubble Heritage Team (STScI/AURA).
The dust starts off as atoms, and the atomic precursors to interstellar dust are carbon and oxygen. Oxygen is twice as common as carbon in the Universe, and is more likely to be produced in supernova explosions, although that is by no means exclusive. Carbon, on the other hand, is a by-product of red giants. Our Sun will evolve into a red giant in about five billion years. To answer the question of whether it will be capable of producing carbon, we need to explore the way in which the chemistry of the Milky Way has evolved over the last 13 billion years.
The first stars only had hydrogen and a little bit of helium to construct themselves from. But what they took from the Universe, they gave back by enriching space with the heavy elements that had forged within themselves.
These first stars were, generally, enormous, probably more massive than almost any star today, and so they exploded as supernovae. This would have produced lots of oxygen, which is the pre-cursor to silicate materials such as olivine. In the beginning, our Galaxy will have been laced with silicate dust – which is good for forming planets – but not much in the way of carbon-based organic molecules and dust, which is crucial for life as we know it.
Dust at high redshifts
Paradoxically, large amounts of dust have been discovered in very distant galaxies 13 billion light years away. These galaxies are all ‘hyperluminous’ infrared galaxies, with star formation rates of 3,000 per year (compare that to the Milky Way’s star formation rate of a measly ten stars per year). As an example, one Galaxy at a redshift of 6.4 was found to contain 200 million solar masses worth of dust, just 890 million years after the big bang.
From what we know about stars and dust production, it is safe to say that a great deal of the dust in very distant galaxies was formed in supernovae, or mass loss from supernova progenitor stars before they exploded, so we can expect the dust to be mostly silicate (although identifying emission and absorption lines at such distances is extremely difficult). However, there’s a problem. Judging by the amounts of dust produced in the nearby Cassiopeia A supernova remnant that exploded about 300 years ago, supernovae alone cannot produce the amounts of dust that is seen in these very young, distant galaxies. This leads astronomers to believe that there must be another production mechanism at work, perhaps in the form of dust accreting directly out of the interstellar medium. There is still much work to be done before we can understand what role dust plays in these galaxies. |
Carbon vs oxygen
However, 13 billion years is a long time, and slowly but surely the balance of power began to shift. It takes longer for stars to become red giants than it does for them to blow up in a supernova, which inevitably led to a delay in the injection of carbon-based molecules into the interstellar medium – the tenuous gas that fills the spaces between the stars. Even then, it is no sure thing that a star will produce carbon.
You might be surprised to find that one of the most common molecules found in stellar outflows is carbon monoxide. It may be poisonous in the home, but out in space it is a vital link in the chemistry chain. This is because it is easy to form, has a high degree of stability in the face of some harsh environments, and contains both one atom of oxygen and one atom of carbon.
In an oxygen-rich star, then any carbon molecules it produces will be swept up inside carbon monoxide molecules, leaving plenty of leftover oxygen atoms spare. But if a star is carbon-rich, then all the oxygen atoms will be locked up inside carbon monoxide molecules, leaving the remaining carbon atoms to go free. This paves the way for the formation of organic molecules such as polycyclic aromatic hydrocarbons, or PAHs as they are known for short.
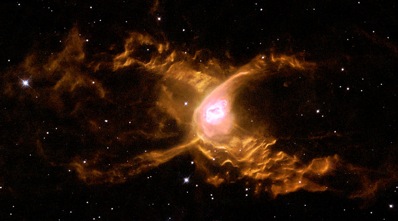
The ‘Red Spider Nebula’ – carbon-based dust is often found in planetary nebulae. Image: ESA/Garrelt Mellema (Leiden University).
The formation of massive stars peaked around eleven billion years ago. As such, the chemistry of the Milky Way Galaxy will have been totally dominated by oxygen-based silicates.
The carbon initially has to be dredged up, via complex internal convection, from deep inside stars that turn into red giants. For stars with zero metallicity (i.e. containing no heavy elements, the first generation of stars), those with a wide range of masses, from just smaller than the Sun at 0.8 solar masses, to 7.8 solar masse giants just under the mass boundary for supernovae, could all dredge up enough carbon to be described as carbon-rich. The most massive of these would begin evolving into red giants after 50 million years. For stars of one solar mass, it takes ten billion years. So you can see where the delay comes in. But as metallicity inside stars rose (the Sun’s metallicity is 0.017, and stars being born today have metallicities of around 0.02, which is still a tiny percentage of a star’s mass), conditions inside the stars changed, making it harder for them to dredge up carbon. And this, over time the range of masses for carbon-rich stars has narrowed to the point today that only stars between 3 and 3.8 solar masses can produce enough carbon. This means that our Sun will not become carbon-rich in the future.
The number of less massive stars vastly overwhelms the number of massive stars destined to become a supernova. Over the aeons, the chemistry of the Galaxy began to alter from purely silicate-based to an increasingly carbon-based one. One of the consequences of all this carbon being added is that dust made from carbon-based molecules is more opaque than silicate dust. Hence, over time galaxies have grown more and more ‘cloudy’, to the point that we cannot readily see the far side of our own Galaxy for the vast sheets of dust between us.
Mixed messages
But how we get from carbon and oxygen atoms, to dust? This is where the environment of the outflow comes into play. Interstellar dust is created when molecules collide and bind together to form tiny grains less than a millionth of a metre in size, which then collide with other grains, creating larger ones. The size and composition of the grains depends hugely on the density of the outflows. The expanding cloud of debris from a supernova is moving rapidly, which doesn’t allow much time for grains to collide and grow bigger before the gas is blown too thinly across space. Conversely, the outflows from red giants, as they puff up and blow off their outer layers, are much more gently in comparison, affording plenty of time for grains to collide and grow and evolve the overall chemical composition.
We do see exceptions to the rule that stars can only be either carbon-rich or oxygen-rich. Sometimes chemical reactions can cause carbon monoxide molecules to split and release their individual atoms. In close binary systems, if one star is carbon-rich and the other oxygen-rich, their shared outer atmosphere will have a mixed carbon/oxygen chemistry. And lately, as reported in the May 2009 issue of Astronomy Now, and also online here some stars near the centre of our Galaxy have been found to have outflows containing not only the likes of carbon-based PAHs, but also silicates. There’s no evidence to suggest that these stars are binaries, so a new explanation has been proposed: that the stars’ helium flash (when temperatures rise hot enough for helium to begin fusion reactions – this can happen several times near the end of a star’s life) causes a thermal pulse that efficiently mixes the carbon and oxygen in the outer layers of the stars.
Currently in our Milky Way there are about 50 million solar masses of interstellar dust floating around, much of it residing within star-forming molecular clouds in the spiral arms. The lifetime for the average dust grain is 500 million years, assuming it isn’t accreted into the planet-building process, which means that the dust is destroyed (via radiation, high temperatures, or shock waves) at a rate of 0.1 solar masses per year. To maintain dust levels, it also has to be replenished at a similar rate. That said, a typical supernova can produce around one solar mass of dust, but as supernovae only occur once or twice a century in our Galaxy, their overall contribution is diminished.
Fifty million solar masses doesn’t sound like much when compared with the trillion plus solar masses of the Galaxy altogether, but just think how many rocky planets could be constructed from that material as it accumulates into bigger and bigger grains in planetary construction yards, eventually forming planetesimals and then fully-fledged planets. Dust in your home is a nuisance; dust in space is amazing, and thankfully there is no cosmic feather duster to wipe it, and consequently us, away.
Further reading
Read the eleven-page Focus on spectroscopy in the May 2009 issue of Astronomy Now, out 16 April, detailing how this astronomical technique informs us of what stars, gas and dust in space are made of.
In the same issue, make sure to read Tracing the Sun’s Family Tree, a four page feature article detailing our hunt for the Sun’s siblings, which formed alongside 4.59 billion years ago, by searching for stars with a similar chemistry to our Sun.
For a more technical write up on the life cycle of interstellar dust, read this paper written by Eli Dwek (NASA Goddard Space Flight Center), Frédéric Galliano (CEA, Saclay, Paris) and Anthony Jones (Institut d’Astrophysique Spatiale, Paris) at the astro-ph website: http://xxx.lanl.gov/abs/0903.0006
The research documented in the paper was presented at the ‘Cosmic Dust Near and Far’ conference in Heidelberg between 8–12 September 2008. |
|
|
|
|
|
|